Star Formation Near and Far
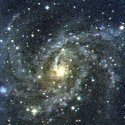
I use molecular spectral line and continuum emission from star formation regions to measure the physical conditions in these regions. The Very Large Array (banner image), 100m Green Bank, IRAM 30m, and Atacama Large Millimeter Array (ALMA) radio telescopes allow me to gather information on the sub- to mega-parsec scale structure of the spatial density, kinetic temperature, and dynamics of these star formation regions.
The most basic of physical parameters which describe galaxies in our universe are in fact the most difficult to determine. A derivation of the density, temperature, and dynamic structure of a galaxy requires sensitive measurements using a variety of atomic, molecular, and infrared emission tracers to devine this most basic information. As these measurements require sampling over a wide range of spatial scales, I use both interferometers and single dish telescopes to gather this information. The primary tool that I use in these measurements is molecular spectroscopy, which is a powerful segment of physics which allows astronomers to derive the physical conditions within the dense gas from which stars form in galaxies.
Star Formation in Galaxies
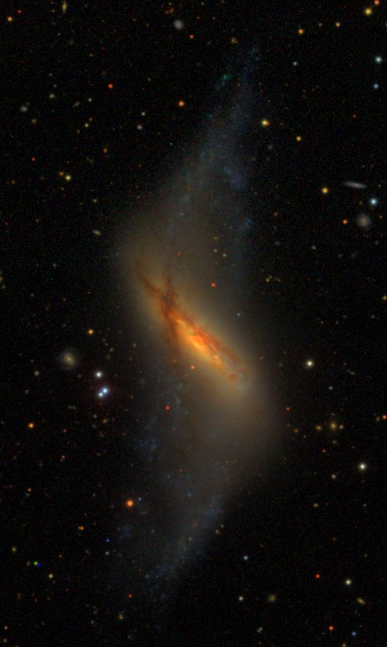
Many galaxies show evidence for having experienced a burst of star formation either very recently or in their not-too-distant past. These starburst galaxies are prime targets for molecular spectral line studies of the star formation process in galaxies. It is also suspected that most galaxies go through this burst of star formation at some point in their evolution. And, to add even more to this mix, there appears to be an interplay between the Active Galactic Nucleus (AGN) phenomenon and star formation which influences the evolution of galaxies. Disentangling the physical process and evolutionary affects which drive this star formation in galaxies is what drives astronomers such as myself to study these objects.
Molecular Spectral Line Diagnostics of the Star Formation Process
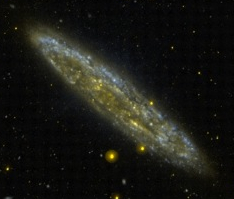
It is a fortunate fact that a wide variety of molecular tracers are detectable in many starburst galaxies. One of the prime examples of starburst activity in the nearby universe is the Sculptor galaxy NGC 253 (at right). The dense gas in these starburst galaxies seems to originate mainly from the nuclei of these objects. In many instances these starburst nuclei show evidence of an interaction, such as by disturbed gas motions or dust lanes (such as the dust lane apparent in the NGC 660 image shown above).
The molecular emission from NGC 253 can be quite strong, an
example of which is shown on the left. Formaldehyde (H2CO) is a
wonderful tracer of the dense gas in star formation regions, and the emission
from the Formaldehyde molecule is really quite strong from NGC 253.
The
spectra shown are from the K-doublet transitions of H2CO, which are
transitions which possess some very useful properties.
Because H2CO is a slightly asymmetric rotor molecule, each
rotational energy level is split by this asymmetry into two energy levels.
Each energy level must then be designated by a total angular momentum quantum
number, J, the projection of J along the symmetry axis for a limiting prolate
symmetric top, K-1, and the projection of J along the symmetry
axis for a limiting oblate symmetric top, K+1. This splitting
leads to two basic types of transitions: the high-frequency ΔJ
= 1, ΔK-1 = 0, ΔK+1 = -1 "P-branch"
transitions and the lower-frequency ΔJ = 0, ΔK-1 = 0,
ΔK+1 = ±1 "Q-branch" transitions, popularly known
as the "K-doublet" transitions. The P-branch transitions are only seen in
emission in regions where n(H2) ≥105
cm-3. The excitation of the K-doublet transitions, though, have
some unique properties. For n(H2) ≤105
cm-3, the lower energy states of the 110-111
through 514-515 K-doublet transitions become
overpopulated due to a collisional selection effect (Evans 1975;
Garrison 1975).
This overpopulation cools the J ≤ 5 K-doublets to excitation
temperatures lower than that of the cosmic microwave background, causing them
to appear in absorption. For n(H2) ≥ 105.0-6.0
cm-3, this collisional pump is quenched and the J ≤ 5
K-doublets are then seen in emission over a wide range of kinetic temperatures
and abundances.